Posted: March 30, 2023
Employing the power of microbes for healthier crops.
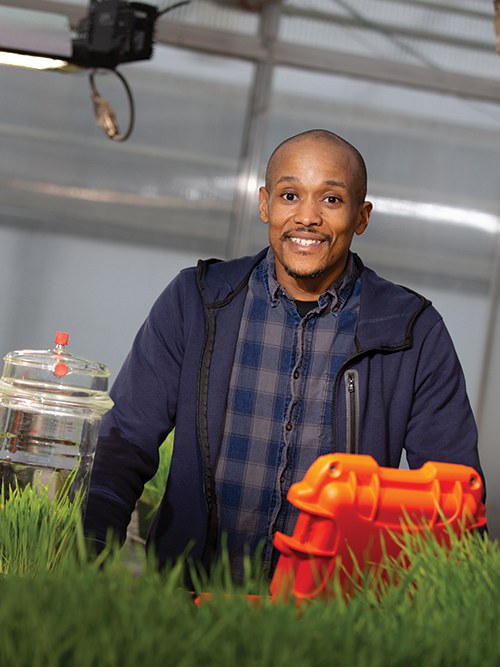
Jarid Ali, associate professor of entomology and director of the Center for Chemical Ecology, combines chemistry and biology to study mechanisms and interactions among insects and plants. For example, plants respond to different stressors by changing their odors.
When it comes to life on Earth, plants make up the bulk of it -- at least according to sheer mass. A 2018 study of life forms on the planet showed that plants account for 80 percent of Earth's total biomass.
But plants can't do it alone. They require an entire ecosystem to survive and thrive. College scientists are exploring how plants interact with the world around them, discovering new interventions to make plants healthier and genetically stronger.
"The potential for plants to offer solutions to some of the most pressing issues of our time lies in their genetic makeup or genomes," said Blair Siegfried, associate dean for research and graduate education and director of the Pennsylvania Agricultural Experiment Station. "An improved understanding of plant genetics offers unique tools to help our scientists breed safe and sustainable varieties of plants that are more productive and can withstand an increasingly unpredictable environment."
Penn State is at the forefront of research in plant genomics. The University has assembled teams around the growing research area with new hires and initiatives, Siegfried noted. "This investment is the launching point for us to bring these technologies to producers, land managers and citizens of our state to provide a safe and nutritious food supply and to maintain a sustainable environment for future generations."
A Dirty Job
On Liana Burghardt's office wall, there's a reproduction of a painting called "Three Sisters in Soil." The painting depicts what indigenous people of North America called the "Three Sisters," a trio of crops (corn or maize, climbing beans or legumes, and squash) traditionally grown together to provide ecological, structural and nutritional balance for one another.
"As evidenced by intercropping systems such as the 'Three Sisters,' humans have known about and leveraged the soil-fertilizing benefits of leguminous plants to improve crop health for thousands of years," said Burghardt, assistant professor of plant science.
Burghardt explained that it was only in the last 100 years or so that scientists discovered bacteria, called rhizobia, living in the plant roots that work to fertilize the "sisterhood" of plants and enrich the soil with nitrogen.
"My lab is thinking about these nitrogen-fixing bacteria from a new angle: Can we leverage the common agricultural practice of planting legume cover crops to enrich soil microbiomes for specific rhizobia that will help fertilize future plants?" Burghardt said.
Liana Burghardt, assistant professor of plant science, is leading a research team studying interactions between legumes and soil bacteria called rhizobia. Her lab is thinking about these nitrogen-fixing bacteria from a new angle.
Burghardt is leading a research team studying interactions between legumes and rhizobia. With a $300,000 grant from the U.S. Department of Agriculture's National Institute of Food and Agriculture, the team is looking at how rhizobial cycling between diverse legumes in crop rotations might affect soil and plant health.
"Legume cover crops are a potentially powerful tool to increase the abundance of specific types of beneficial bacteria for the downstream legume cash crops -- but no one has ever measured this possibility," Burghardt said.
Leveraging a long-term field experiment at Penn State's Russell E. Larson Agricultural Research Center at Rock Springs, the team is sampling the roots of different legume cover crops and cover crop mixtures and then using genome sequencing to measure the rhizobia associated with those cover crops. Then they'll analyze the rhizobia associated with the cash crops, such as soybeans, later planted in the same fields as the cover crops.
Burghardt also received a three-year, $650,000 USDA-NIFA grant to lay the groundwork to breed for "mutualisms" by tracking the long-term adaptation of nitrogen-fixing rhizobia in agricultural fields. Mutualisms describe ecological interactions between organisms from which both organisms benefit -- in this case, rhizobial soil bacteria gain sugars and leguminous plants gain nitrogen.
"Legumes establish beneficial associations with rhizobia that perform biological nitrogen fixation, but this mutualism often doesn't work well in agricultural systems," she explained. "Most seeds are sold already coated with rhizobial bacteria, but those inoculants often fail to survive or compete in agricultural soils."
Burghardt and her team are using alfalfa variety trials at Penn State research farms to measure how various species of alfalfa plants influence microbial evolution in the soil. "We look at the nitrogen-fixing nodules on alfalfa roots that house bacteria to measure how rhizobia adapt to alfalfa varieties across seasons and years," she said. "To do this, we've been going out to the field to dig up nodules in the spring and fall from multiple alfalfa varieties across sites and planting years."
Because alfalfa plants are perennial and the variety trial plots have been in place for five years, the plants have a long history of forming relationships with bacteria and releasing them back out into the soil year after year, creating the opportunity for the plants to influence microbial evolution in the soil.
"It's exciting because no one has ever measured these dynamics before," she said. "For obvious reasons, we've focused on the plant perspective -- how are the microbes benefitting the plants. But let's see how the plant is influencing microbial evolution, because it's the plant that will determine what microbes are present in the soil for the next plant and the next plant and the next plant. If we can harness the power of host-microbiome manipulation to develop cultivars that are great at selecting helpful bacteria, we can promote efficient nitrogen fixation that can lead to more robust yields and better soil health."
Fundamental Fungus
Ruairidh Sawers, assistant professor of plant abiotic stress, also is interested in soil microbiomes -- those that interact with corn. In his maize genetics lab, he and his team are using DNA sequencing to analyze communities of soil fungi and how they interact with corn plants, with the aim of boosting crop resilience and production.
With a USDA-NIFA grant of nearly $1.25 million, the researchers want to determine which corn varieties and which corn genes promote the greatest benefit from the most common symbiotic association of plants with microbes, the root-based arbuscular mycorrhizal (AM) fungi.
"Mycorrhizal fungi have been fundamental from the very beginning of plants on land, and they remain ubiquitous in our major cereal crops," Sawers explained.
Ruairidh Sawers, assistant professor of plant abiotic stress, and his team are using DNA sequencing to analyze communities of soil fungi and how they interact with corn plants, with the aim of boosting crop resilience and production.
Although arbuscular mycorrhizal fungi are present in cultivated corn fields, it's unclear how much they contribute to productivity, he added. To address this question, Sawers and his research team have generated corn hybrids that no longer interact with mycorrhizal fungi.
"By growing regular corn and corn that cannot associate with the fungus side by side, we can ask how much the variety without this fungus is losing out and infer how important the fungus is normally," Sawers said.
The researchers are seeing that, for many given field conditions, the impact of mycorrhizal fungi is considerable, and that some corn varieties respond better to mycorrhizal fungi than others.
"So, the big question is, how and why? We're studying this at the genetic level because we want to understand which bits of the genome are important to driving these differences," said Sawers.
In field trials at the Russell E. Larson Agricultural Research Center, Sawers and colleagues are making further use of their nonmycorrhizal varieties in genetic mapping experiments.
"We're looking at plants and soil at the physiological level," he said. "On one end of the spectrum, measuring very simple things, like how heavy is the ear of corn -- all the way to characterizing the entire microbiome community."
The experiments will distinguish plants that perform poorly without the fungi from those that show an exceptional capacity to benefit from the symbiosis.
"We're interested in the trade-offs between the performance of symbiotic and nonsymbiotic plants to determine the extent to which breeding for high-input agriculture can also be aligned with maintaining the capacity of plants to benefit fully from mycorrhizal fungi associations," Sawers said. Plants and fungi have co-evolved over 450 million years, he added.
"They developed a balance, a symbiotic interchange, in which both the plant and the fungi benefitted," he said. "But when we began to domesticate plants, and then using industrial fertilizer on fields, that balance changed dramatically. Our current high-input agricultural environment is vastly different from the environment in which these plants evolved."
The prevailing hypothesis that agricultural intensification has reduced the benefit modern crops can derive from microbial interactions is fundamental to understanding plant-microbial associations, but it has been difficult to address, Sawers explained.
"Manipulating microbial communities in the field at scale is logistically challenging," he said. "But if we can better understand when, why and how plant-microbial interactions occur, we'll be closer to fully realizing their potential in agriculture."
Fighting Microbes With Microbes
While researchers dig deep to learn more about the soil microbiome, plant pathologist Kevin Hockett focuses on the phyllosphere: the above-ground portion of plants that includes stems, leaves, flowers and fruits.
Hockett, assistant professor of microbial ecology and Lloyd Huck Early Career Professor, received a $682,500 USDA-NIFA grant to study bacterial control methods and their implications for disease prevention in plants.
It's well known that microbial communities in the soil can suppress plant diseases, Hockett explained, offering an example of a disease-sensitive wheat variety. If that variety has a certain level of a pathogen one year, it will have a higher level the next year -- with disease levels increasing each year because more and more of that pathogen is built up in the soil.
Kevin Hockett, assistant professor of microbial ecology and Lloyd Huck Early Career Professor, is studying microbial communities and bacterial control methods and their implications for preventing and suppressing plant diseases.
"Eventually, you reach a peak, and then the disease starts to come back down naturally," he said. "As their populations increase, certain bacterial populations in the soil start to inhibit the pathogen, acting as a natural biocontrol. This is really exciting, but it's hard to take advantage of that as a grower because a grower can't take five to seven years suffering greater losses each year."
While the phenomenon of disease-suppressive soils is generally understood, there has never been a description of a disease-suppressive community in the phyllosphere, Hockett said.
"That's probably because you don't have this microbial community in the environment year after year," he said. "With tomatoes, for example, you grow the plants and harvest them. Those plants go away, but all the ecological and evolutionary dynamics occurring in the soil continue to play out. The above-ground portion of the plant gets only one season, with the harvest acting as a sort of reset button."
Hockett and former doctoral candidate Hanareia Ehau-Taumaunu wondered what would happen if they tried to force disease-suppressing dynamics in tomatoes over several growing cycles. In a greenhouse study of young tomato plants representing different treatments and control groups, they repeatedly "passaged," or transferred, microbes -- initially gathered from leaves of healthy tomato plants in the field -- from one plant to another. They then inoculated tomato plants with the bacterial speck pathogen.
For 10 cycles, the researchers looked for plants that had the least amount of disease. The plants with the lowest disease severity were then selected as the microbial community source in the next round of inoculation. The idea of successive passaging of the microbial community between tomato plants essentially served to speed up ecological and evolutionary processes, simulating the season-to-season transfer of microbes repeatedly exposed to bacterial speck.
After multiple transfers of the microbes, disease symptoms such as brown or black lesions on leaves began to wane and, by the ninth passage, declined by nearly half.
"That's what we were hoping we'd see," Hockett said. "The disease buildup in the phyllosphere ultimately resulted in disease suppression as a normal ecological response to pathogen pressure. This was the first study to show that microbial communities could play a similar role in the phyllosphere. We don't know exactly how they're working, but we do know that going through this process leads to the development of a community that is now suppressing disease."
This discovery offers hope that growers someday can apply mixtures of bacteria and fungi to protect plants and improve harvests.
"Our goal is to develop a process that enables growers to use microbial communities in the field, and this work has implications beyond tomato and even vegetable plants," Hockett said. "I'd like to work with collaborators to see if we could get this to work with fungal pathogens as well as other bacterial diseases in other vegetable crops."
It's All Connected
Entomologist Jared Ali shares that enthusiasm for collaborating with colleagues to better understand complex chemical interactions and improve plant health. Ali, associate professor of entomology and director of the Center for Chemical Ecology, combines chemistry and biology to study mechanisms and interactions among insects and plants.
All organisms interact with, and are changed by, the molecules around them, Ali explained.
"We're in this chemical mixture of odors and pheromones and scents and molecules that change us and our behavior every day -- from the food we smell cooking to the smoke from a fire to the aroma of blooming flowers," he said. "All these things have messages and purposes, and part of the goal of my research is to understand which cues we can use to manipulate systems around us."
Plants respond to different stressors by changing their odors: One of the simplest examples of this phenomenon is the scent of newly cut grass, which is produced by volatiles released by damaged plant tissue after mowing. Ali and his colleagues use volatiles, or odors, to better understand a plant's condition and to manipulate chemicals in plants to deter pest insects or attract beneficial organisms.
"So instead of dumping pesticides on a plant to protect it, you could use odors that make that plant less preferable or less attractive to pests," he said.
In addition to above-ground stressors, plants also respond to changes in the soil, becoming more or less susceptible to particular pests due to different combinations of soil microbes, Ali explained.
In corn research plots at the Russell E. Larson Agricultural Research Center, Ali and his research team sampled odors in corn plants grown in soil that previously hosted cover crops, such as wheat and radish, to look for chemical changes and gauge whether those changes affected cash crop susceptibility to insect predators.
"This cover crop work is exciting because we're tapping into a tactic farmers are already using. Cover crops do a lot of great ecosystem services to maintain soil health, but now we can link something growers are using to chemical ecology signatures," Ali said, noting that this work can lead to recommendations for cover crops that could help cash crops resist specific pests or pathogens.
Ali relies on collaborations across the college, noting that the Center for Chemical Ecology helps him build connections.
"I have colleagues in plant pathology who tell me which pathogens I should be concerned with," he said. "For example, certain pathogens produce toxins in grains that could be dangerous for humans and livestock. If we could use odor to recognize those toxins, we could prevent damaged grain from getting to market. I also count on our friends in Penn State Extension to keep us reeled in, letting us know what issues the farmers are dealing with and what they're worried about."
Promising Frontier For Research
Scientists in the college are at the forefront of extraordinary and innovative research in plant genomics, and the future could look even brighter with the prospect of enhancements to labs and greenhouse facilities.
"The college's research programs are a critical concern, and we are working to garner funding to modernize some of our plant research facilities," said Rick Roush, dean of the college. "Our faculty and students can do so much more with enhanced facilities, which are critical if the college is to continue attracting and retaining top researchers who will develop solutions that will benefit both growers and consumers."
By Krista Weidner and Jeff Mulhollem
Photography by Michael Houtz
Features
Fostering Forests
Across the United States, forests face unprecedented threats, and scientists in Penn State's College of Agricultural Sciences are conducting novel and complex research to conserve them.
Buzzing With Purpose
Community scientists work to protect Pennsylvania's wild bees
Conservation Reimagined
Exploring new approaches to cope with a changing climate